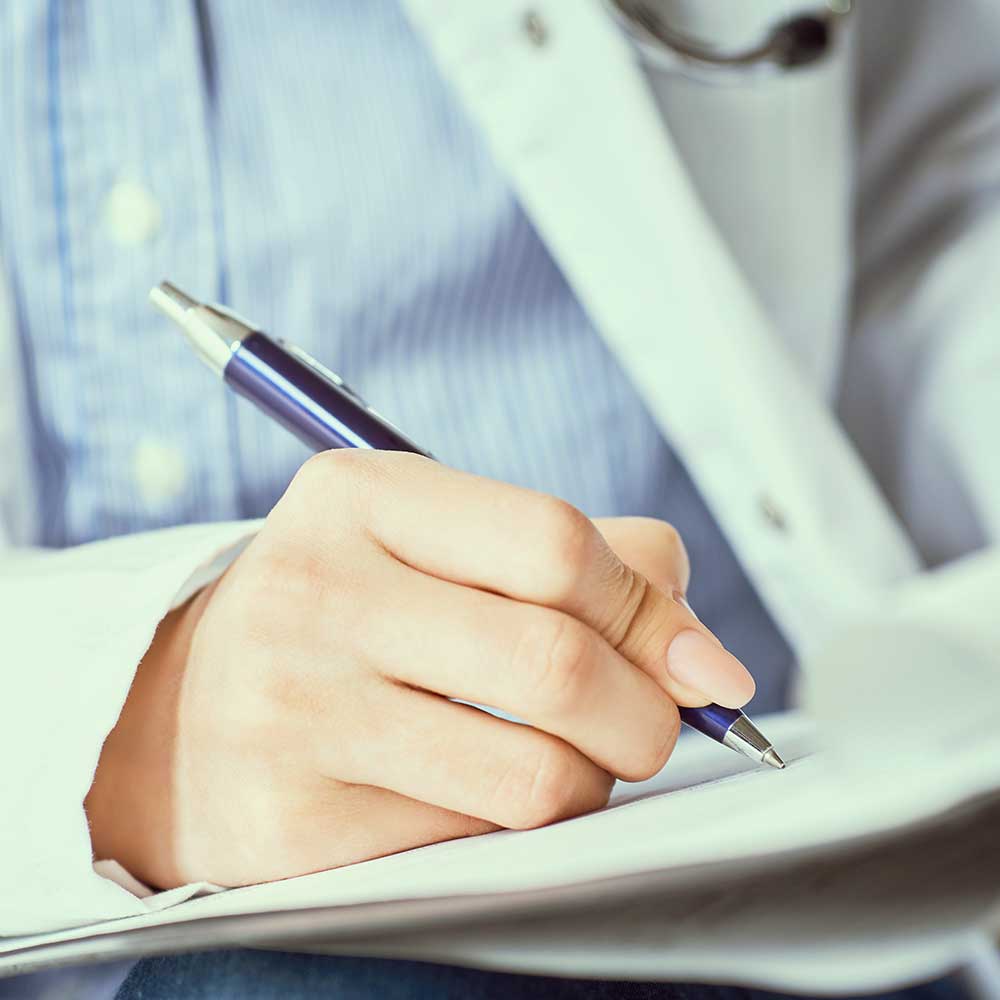
Introduction
By June 15th 2020, 7,997,084 people were infected with SARS-CoV-2, and 435,662 had died. The world economic loss is estimated to be at least $1 trillion. In response, multiple vaccine trials are underway, but none are expected to be out of phase 3 trials until mid-2021.
This delay has necessitated the reassignment of several drugs from their original treatment purpose so as to rebuild the antiviral armamentarium. One of these redirected drugs is hydroxychloroquine (HCQ), currently approved in Australia for discoid and systemic lupus erythematous, rheumatoid arthritis and the suppression and treatment of malaria.
Early trials with HCQ were equivocal at best, forcing clinicians to balance the possibility of clinical benefit against the cardiotoxic effects in vulnerable patients with multiple comorbidities.
In mid-May 2020, three significant papers were released which add more certainty to the use of HCQ, or its precursor, chloroquine (CQ) in COVID-19 (C-19) patients.
On May 22nd 2020, in The Lancet, Mehra and co-workers reported the results of an international, observational registry analysis involving the use of chloroquine or hydroxychloroquine with or without a macrolide (azithromycin or clarithromycin) between 20 December 2019 and 14 April 2020, in COVID-19 patients across 671 hospitals. There were 96,032 patients in the study. Of these, 1868 received chloroquine only, 3783 received chloroquine with a macrolide, 3016 received hydroxychloroquine only, and 6221 received hydroxychloroquine with a macrolide. There were 81,144 patients in the control group.
The mean daily doses of the various drug regimens were: chloroquine alone – 765mg; hydroxychloroquine alone – 596mg, chloroquine with a macrolide – 790mg; and hydroxychloroquine with a macrolide, 597mg.
After controlling for confounders, the researchers reported that the mortality in the control group was 9·3%, in the hydroxychloroquine group it was 18·0%, in the hydroxychloroquine plus macrolide group it was 23·8%, in the chloroquine alone group it was 16·4%, and in the chloroquine with a macrolide cohort is was 22·2%. Each treatment modality was independently associated with an increased risk of in-hospital mortality.
Commenting on the Lancet study, Dr Josh Davis of the Doherty Institute and principal investigator of the ASCOT (hydroxychloroquine) study planned by the Institute noted that patients in the Lancet paper who received HCQ “ … had a higher risk of death at baseline than those who didn’t. They were sicker and they had more comorbidities. That alone could potentially explain the higher risk of death. The only way of knowing whether that’s true or not is to do a randomised trial.”
This concern is well-founded because the co-administration of CQ/HCQ and a macrolide is known to cause an increased corrected QT interval. A prolonged QT interval (the time required for the ventricles to depolarise and repolarise) can precipitate ventricular arrhythmias such as torsades de pointes, which can spiral into ventricular fibrillation.
The Subsequent Controversy
On 23 May 2020, one day after publication of the Mehra paper, the World Health Organization (WHO) paused patient recruitment into the hydroxychloroquine arm of the SOLIDARITY trial, citing safety issues with HCQ that were highlighted by Mehra et al.
The SOLIDARITY study is an adaptive, randomised, open-label, controlled clinical trial, in collaboration with countries around the world through the World Health Organization.
Subjects will be randomised to receive either standard-of-care products or the study medication plus standard of care, while being hospitalised for COVID-19.
Participants will be randomised to one of the following groups:
- Lopinavir/ritonavir 400mg/100mg orally twice daily for 14 day plus local standard care, OR
- Hydroxychloroquine 800mg twice daily for 1 day then 400mg twice daily for 10 days plus local standard care, OR
- Remdesivir 200mg IV on day 1, followed by 100mg IV daily infusion for 9 days plus local standard care, OR
- Lopinavir + ritonavir (orally twice daily for 14 days) plus interferon-beta (daily injection for 6 days). OR only
- Local standard support care.
“The patients will be followed up for the entire length of their hospital stay. Death from any cause will be recorded and this will be the main result used to determine whether a drug is effective. Length of hospital stay and time to first receiving ventilation (or intensive care) will also be recorded and used to determine the drug’s effectiveness.”
By 28 May 2020, there was a swift international response to the Lancet study, with 201 scientists co-signing an open letter to the study authors and Mr Richard Horton, Editor of The Lancet. They cited ten concerns including the non-release of data source codes, no ethics committee review, mean daily doses of hydroxychloroquine that are 100mg higher than FDA (US Food and Drug Administration) recommendations, inadequate allowance for confounders, and more technically, “the tight 95% confidence intervals reported for the hazard ratios appear inconsistent with the data. For instance, for the Australian data this would imply about double the numbers of recorded deaths as were reported in the paper.” The authors also denied a request for information on the contributing study centres.
On 29 May, the authors published a correction pertaining to the countries of origin for some of the data. They noted: “the numbers of participants from Asia and Australia should have been 8101 (8·4%) and 63 (0·1%), respectively. One hospital self-designated as belonging to the Australasia continental designation should have been assigned to the Asian continental designation… The unadjusted raw summary data are now included. There have been no changes to the findings of the paper. These corrections have been made to the online version as of May 29, 2020, and will be made to the printed version.”
On 3 June 2020, the Editor of The Lancet issued an Expression of Concern letter, due to serious questions pertaining to the integrity of the data supplied to the researchers by Surgisphere, a company started by Dr Sapan Desai, one of the authors of the Mehra paper.
On the same day the Director-General of WHO announced that on the basis of the available mortality data, the Executive Group of the Solidarity Trial had agreed to the continuation of all arms of the Solidarity Trial, including the hydroxychloroquine arm, as there was “no reasons to modify the trial protocol”.
Finally, on 4 June 2020, the Mehra paper was withdrawn by three of the authors. In the notification published by The Lancet it was stated the authors “… were unable to complete an independent audit of the data underpinning their analysis. As a result, they have concluded that they “can no longer vouch for the veracity of the primary data sources.” The Lancet takes issues of scientific integrity extremely seriously, and there are many outstanding questions about Surgisphere and the data that were allegedly included in this study.”
In a further twist to the SOLIDARITY trial, on June 17th the WHO announced that they were reversing the decision to reinstall the HCQ arm of the trial, and were cancelling further enrolment of patients in this arm.
The decision was predicated “on evidence from the Solidarity trial, UK’s Recovery trial and a Cochrane review of other evidence on hydroxychloroquine.
Data from Solidarity (including the French Discovery trial data) and the recently announced results from the UK’s Recovery trial both showed that hydroxychloroquine does not result in the reduction of mortality of hospitalised COVID-19 patients, when compared with standard of care.”
Patients already taking HCQ will either complete their course or stop at the discretion of the supervising physician.
“This decision applies only to the conduct of the Solidarity trial and does not apply to the use or evaluation of hydroxychloroquine in pre or post-exposure prophylaxis in patients exposed to COVID-19.”
Mahévas research
On May 22nd, 2020, Mahévas and others (BMJ) published the results of a comparative observational study of patients (n = 181) hospitalised between March 12-31, 2020. The study cohort was aged 18-80 years with documented SARS-CoV-2 pneumonia who required oxygen but not intensive care (IC).
The treatment group (n=84) received HCQ 600mg per day within 48 hours of admission, another eight patients received HCQ more than 48 after admission, and the control group (n = 89) did not receive HCQ. The authors concluded that the use of HCQ in COVID-19 patients admitted to hospital requiring oxygen was not supported by the evidence.
Brian J Lipworth, Professor of Pulmonology (Scottish Centre for Respiratory Research, Ninewells Hospital and Medical School) noted that 85% of patients in this study who were given HCQ had evidence of the cytokine storm because their C-reactive protein (CRP) levels were greater than 40mg/L on admission. A CRP higher than 42mg/l on admission is predictive of a poor outcome.
Hence, there was no clinical merit in treating 85% of these patients with HCQ – they were already in the storm phase. The putative mechanisms of action of HCQ-only therapy include interference with viral binding to the angiotensin-converting enzyme II (ACE2) pulmonary receptor, thus reducing viral-cell membrane fusion, and increasing intracellular pH, thereby reducing viral replication.
The presence of the cytokine storm of interleukin-6 (IL-6) means that HCQ use is clinically too late. Only drugs such as tocilizumab, an IL-6 receptor blocker, have clinical justification at this point in the disease progress.
Carlucci research
The third paper in May 2020 was by Carlucci and co-workers who conducted an observational study on patients hospitalised at four New York hospitals between March 2nd 2020 and April 5th 2020, using the triple therapy of hydroxychloroquine (HCQ), azithromycin (AZM) and zinc sulfate (Zn) compared to HCQ plus AZM only use (pre-print, not yet peer-reviewed).
In the triple therapy arm, 411 patients had received hydroxychloroquine 400mg as a loading dose, then 200mg twice a day for five days, zinc sulfate 220mg (containing 50mg elemental zinc) twice daily and azithromycin 500mg daily.
In the comparator arm, 521 patients took HCQ plus AZM only, at the same treatment level. All patients received usual supportive care.
Univariate analyses revealed that zinc sulfate increased the frequency of discharges home, and decreased ventilation need, ICU admissions, mortality or transfer to hospice for patients who were never admitted to the ICU.
After adjusting for the time at which zinc sulfate was added, there remained a significant increased frequency of being discharged home, a reduction in mortality and a reduction in hospice transfer.
There are several caveats to note. First, this study is still in the pre-peer review stage. Second, it was, like those of Mahevas and Mehra, a retrospective, observational study, leading to many potential confounders influencing the results. Carlucci noted the following:
- There was uncertainty as to when each medication was given (as distinct from when it was charted);
- The time when each group commenced their therapy may have differed;
- They were uncertain if the observed added benefit of zinc sulfate to hydroxychloroquine and azithromycin on mortality would have been seen in patients who took zinc sulfate alone or in combination with just one of those medications;
- This study should not be used to guide clinical practice; and
- The point in clinical disease status at which patients received those medications could have differed between our two groups.
The difference in the S and R forms of hydroxychloroquine
In a pre-print paper (not yet certified by peer review), Guanguan Li and co-workers have reported that in all clinical trials, chloroquine diphosphate or hydroxychloroquine sulfate have invariably been used. These formulae are a 50/50 racemic mixture of the R- and S- forms. However, their laboratory tests have shown that there are stereoselective differences in the respective potencies of the R- and S-enantiomer for both chloroquine and hydroxychloroquine against live SARS-CoV-2.
“S-chloroquine (S-CQ) and S-hydroxychloroquine (S-HCQ) were found to be 27% and 60% more active against SARS-CoV-2, as compared to R-CQ and R-HCQ, respectively.”
Added to this is the knowledge that “S-chloroquine seems to be less toxic than the R-chloroquine.” By extension at least, it is reasonable to conclude that S-hydroxychloroquine is less toxic than R-hydroxychloroquine, though clinical trials would need to verify this assumption.
Zinc, immune function and antiviral properties
This study poses an interesting clinical question – why was there a benefit in the zinc arm of the review?
Age is a significant factor contributing to C-19 mortality. A Chinese study (n =72,314) reported that whilst the overall mortality rate was 2.3%, the rate increased proportionally with age reaching 8% in those aged 70–79 years and 14.8% in those ≥80 years old.
One possible contributing factor to the age-related death rate is evidence showing a decline in immune function with advancing years, known as immunosenescence, with studies suggesting an impaired T-cell function against pathogens. Zinc is a contributor to functional immune capacity, with a deficiency causing a rapid reduction in innate and acquired immunity. Hence zinc is considered an essential antiviral micronutrient.
The evidence points to zinc’s antagonistic role in viral transcription. Replication of all positive-strand RNA viruses, such as SARS-CoV and SARS-CoV-2, require membrane-embedded RNA-dependent RNA polymerase (RdRp). This enzyme is at the core of the viral RNA replication machinery. RdRp catalyses the replication of RNA from RNA templates.
Hence the importance of research by te Velthuis and co-workers who have shown that zinc inhibited SARS-CoV RdRp elongation. When compared to alternate metal ions such as cobalt or calcium, zinc was the most effective inhibitor. Zinc caused a 3-4 fold reduction RNA binding to the assay template.
Figure 1. Increasing zinc (Zn2+) concentration causes a decreasing level of RNA1, due to declining RdRp activity (from te Velthuis et al.)
However, it is clinically challenging to achieve an antiviral intracellular concentration of zinc because it is bound to metallothioneins.
The answer to increasing the intracellular zinc levels is to use an ionophore, a chemical species that carries ions across a cell membrane before releasing the species into the cytoplasm. Te Velthuis achieved RdRp inhibitory levels of zinc by using pyrithione (PT) as the ionophore – Carlucci used hydroxychloroquine.
It is important to note that te Velthuis tested zinc against SARS-CoV, whereas Carlucci tested it against SARS-CoV-2, another member of the coronavirus family. Because the amino acid sequence identity between SARS-CoV and SARS-CoV-2 S-proteins is 76.47%, it is clear that these two viruses are genetically closely related, hence the efficacy of zinc against both species. Reinforcing their commonality is that their access into a host cell uses the same cell surface receptor – ACE2.
Conclusions
Zinc is a vital immune-related micronutrient and possesses dual antiviral activity. It enhances the activity of both the innate and acquired immune system and impedes RNA-dependent RNA polymerase (RdRp). HCQ also has a putative duality of antiviral action. It interferes with the ACE2 receptor, potentially impeding ACE2/SARS-CoV-2 binding, and it has been shown to increase the endosomal pH, which may impair SARS-CoV-2 early-stage viral replication.
Triple therapy for five days of HCQ 400mg immediately then 200mg twice daily, AZM 500mg daily and zinc sulfate 220mg – containing 50mg elemental zinc – twice daily has shown clinical benefit in non-ICU bound patients. These results are yet to be confirmed by peer review.
Finally, the use of HCQ in patients with a high CRP is without clinical justification.